In 2006, Dr. Shinya Yamanaka and Dr. Kazutoshi Takahashi changed the world. If both stem cells and somatic had a full set of DNA, they hypothesized that they could manipulate the adult cells to revert into stem cells.
The two chose 24 genes associated with pluripotency. They introduced these genes to adult mouse fibroblast cells using retroviruses as molecular couriers to deliver genetic material to the cells.
They looked for cells that had begun re-expressing Fbx15, a gene expressed solely in embryonic stem cells. This gene is usually silenced in mature cells.
Through this experiment, they narrowed the 24 factors down to Oct4, Sox2, Klf4, and cMyc. These are collectively known today as the Yamanaka factors.
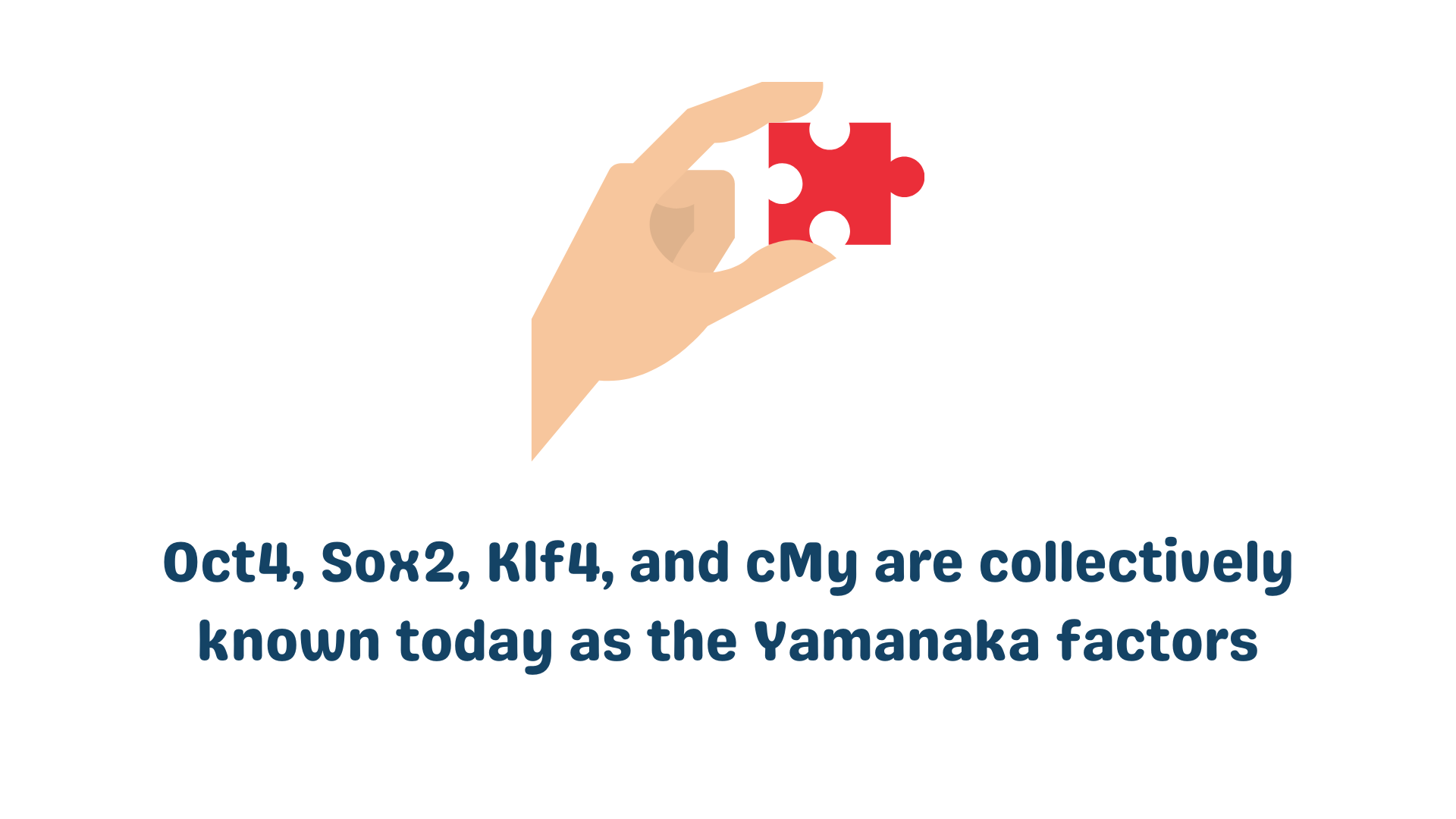
This is where induced pluripotent stem cells come into play.
But what is an induced pluripotent stem cell?
What are stem cells?
To fully understand the definition of an induced pluripotent stem cell, we first have to understand the definition of stem cells in general.
A stem cell is an undifferentiated cell that can divide to produce more stem cells. These are cells that can then change into other types of cells. Scientists call this process “differentiation”, or the process of a cell becoming specialized.
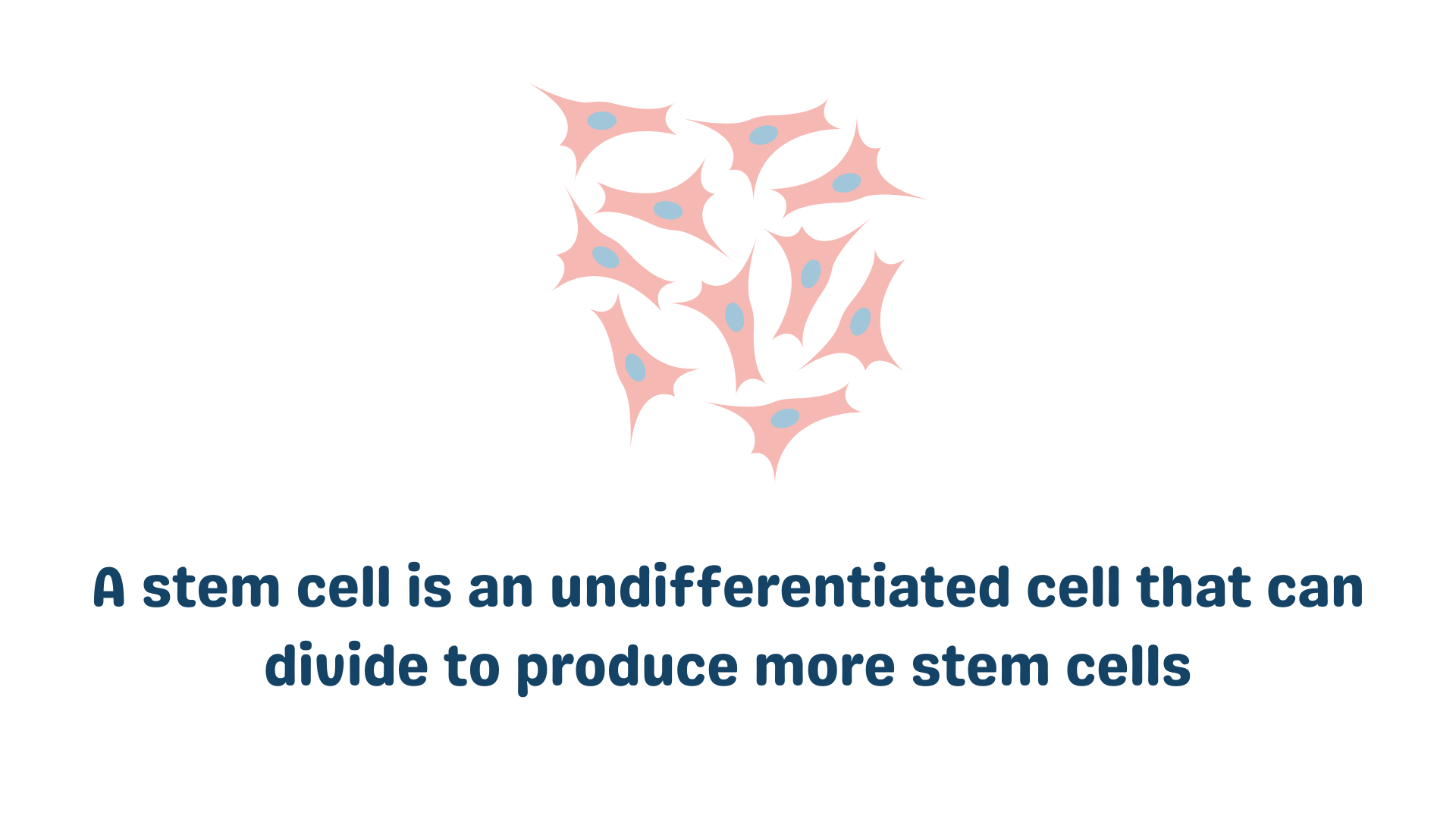
Stem cells are the root source of all the cells in the body. They transform into cells that create tissues and organs for both plants and animals.
Stem cells occur naturally in two ways: embryonic stem cells and adult stem cells. Adult stem cells are also called tissue stem cells.
However, there is a way to artificially create stem cells. We call these induced pluripotent stem cells.
What does “pluripotent” mean?
Stem cells go through several stages of transformation to become muscles, bones, neurons, and organs.
Embryonic stem cells transform into other kinds of stem cells. This second generation then transforms into a third generation. As mentioned before, scientists call this differentiation.
Eventually, the stem cells transform into the final product: you.
As stem cells differentiate, their ability to become anything decreases. They become more specialized. In other words, with each transformation, there are less options for what the “end product” can become.
This phenomenon is sometimes described as “losing potency”. This means that there are different levels of potency, one of which is pluripotent.
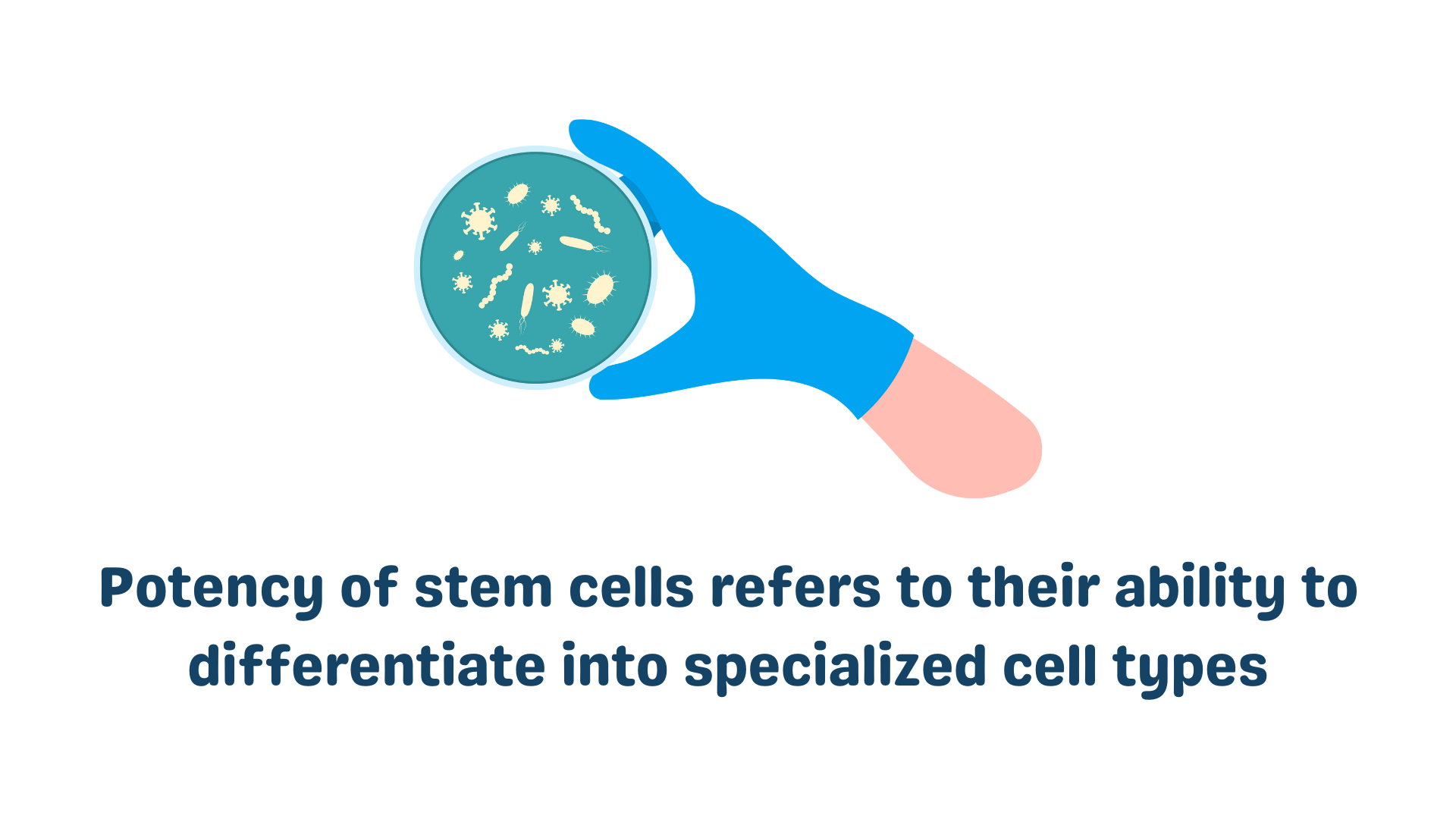
So, what are the different types of potency and what does pluripotent mean?
Totipotent Stem Cells
Totipotent cells can form all the cell types in a body. They form extraembryonic cells, which transform into a baby’s placenta. They also form embryonic cells, which transform into the baby.
In other words, totipotent cells can continue dividing and differentiating to form an entire organism.
Pluripotent Stem Cells
Pluripotent stem cells turn into any type of cell that makes up the body. This is different that totipotent stem cells because they do not form the placenta.
Pluripotent cells on their own cannot form an entire organism because the placenta is an integral part of creating new life.
Multipotent Stem Cells
Multipotent cells are the most limited in their differentiation potential. These stem cells are precursors to specific cell groups that exist in adult organisms. They are responsible for maintenance and repair.
For example, hematopoietic stem cells are a type of multipotent stem cell that multiply and differentiate into different blood cells. Hematopoietic stem cells can never become neurons or organs.
What is an Induced Pluripotent Stem Cell?
Induced pluripotent stem cells, often referred to as iPSCs, are a type of stem cell that used to be adult stem cells. When chemistry meets biology, scientists can transform these cells back into pluripotent stem cells.
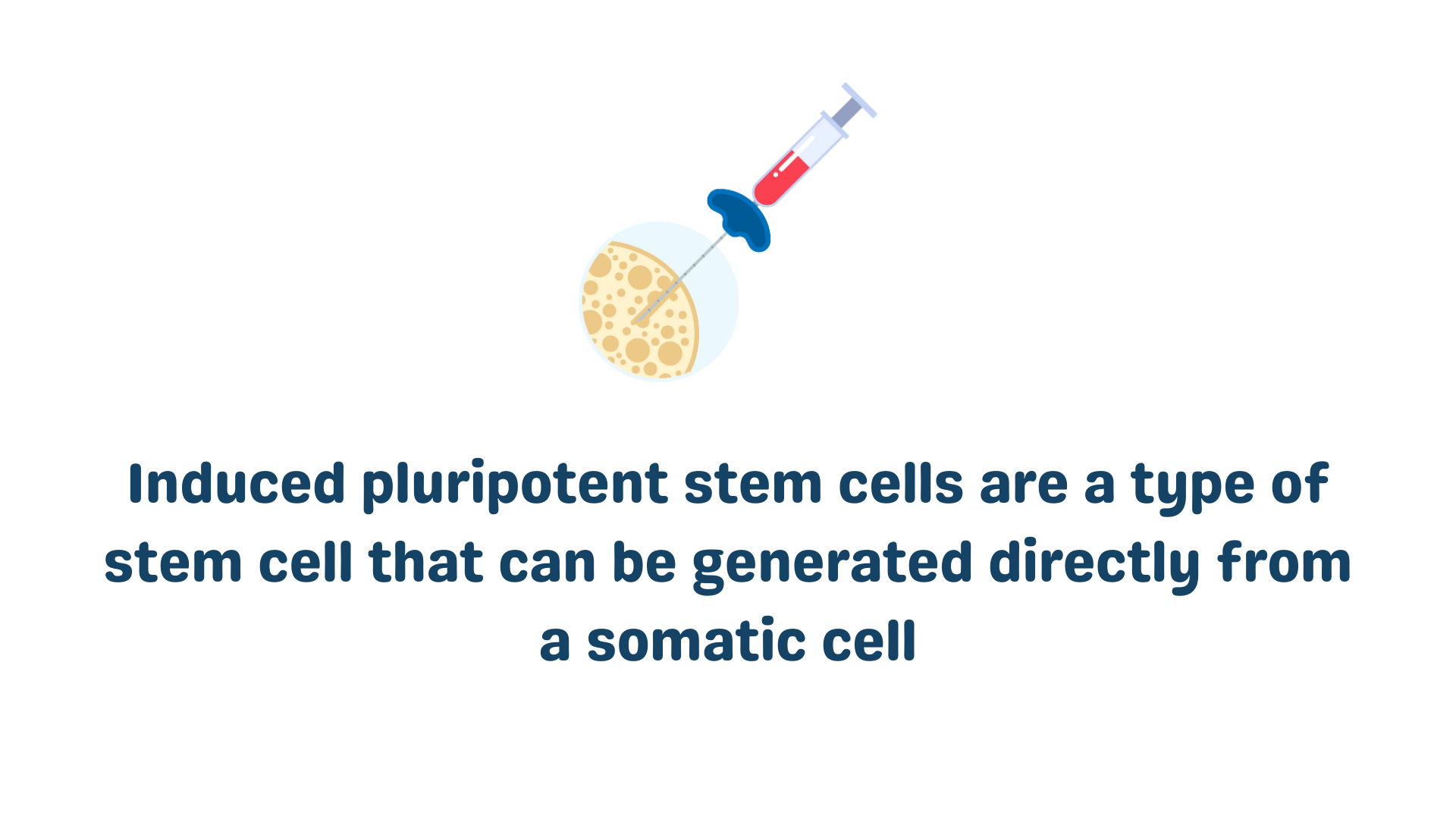
In other words, scientists transform these cells to once again turn into nearly any cell in the human body.
How do they do this?
Essentially, a group of Japanese scientists discovered a way to reprogram cells back to their pluripotent state.
From this “induced” pluripotent state, scientists could “expose” the iPSCs to other factors and differentiate them into nearly any cell.
Now, instead of having to extract cells from blastocysts (the part of the embryo that becomes the fetus), scientists could just take somatic cells and convert them into iPSCs
What are iPSCs used for?
Harnessing the capabilities of iPSCs opens a whole world of possibilities.
Since their discovery in 2006, more and more researchers are turning towards iPSCs as a tool for research and medicine.
A variety of companies and institutions are harnessing the potential of iPSCs to meticulously model an extensive array of diseases. These include but are not limited to…
- Alzheimer’s disease
- Various heart conditions
- Kidney disorders
- Muscle ailments
- Blood-related illnesses
- Diabetes
iPSCs are vital for advancing our understanding of diseases, developing new therapies, and providing personalized approaches to medicine. The versatility and potential of iPSCs make them a cornerstone in modern biomedical research and regenerative medicine efforts.
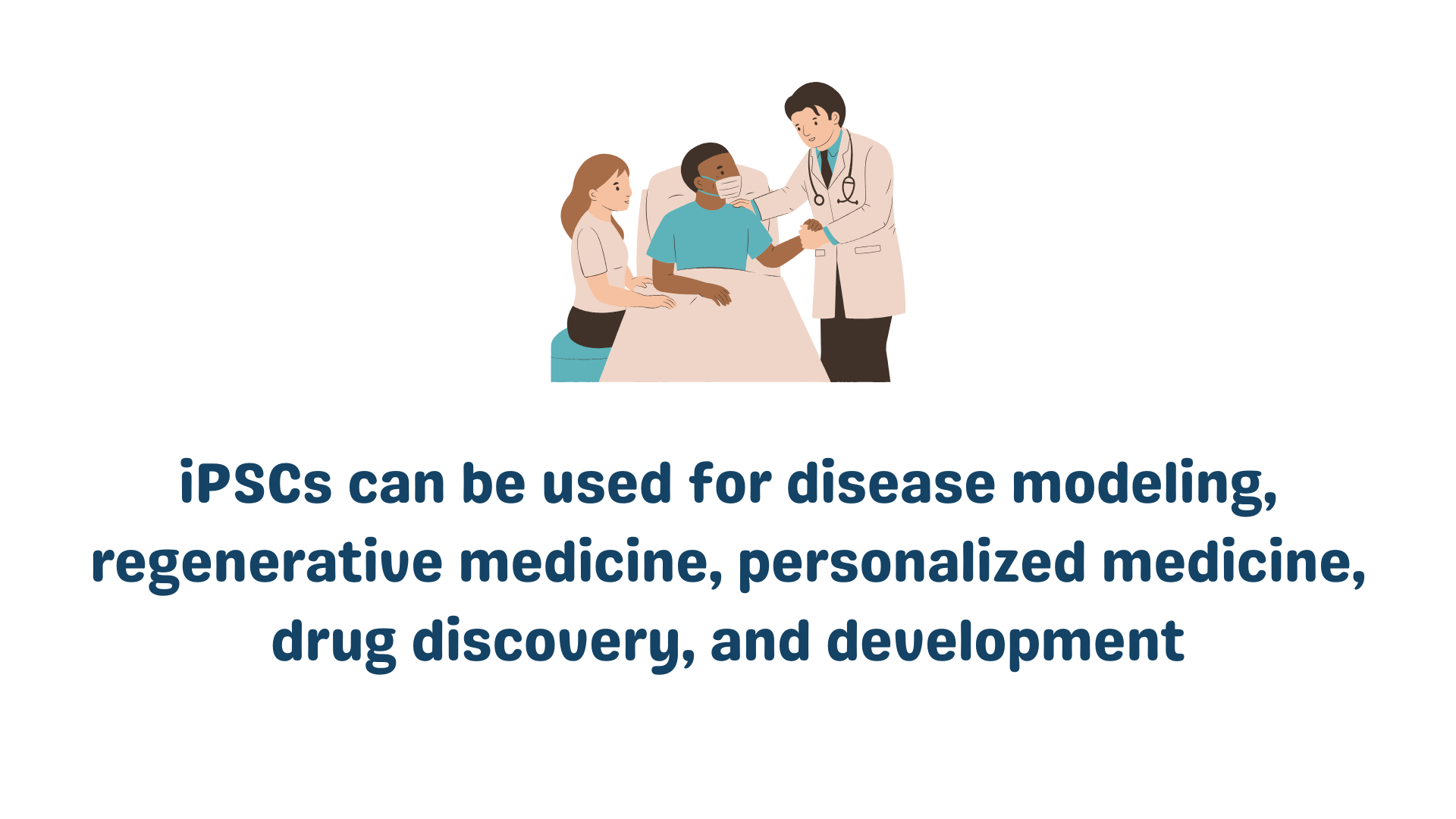
Here are some ways researchers are using iPSCs to better the world:
Disease modeling
iPSCs provide a way to model diseases and test treatments.
Scientists can create iPSCs derived from patients with genetic disorders. This allows scientists to study disease mechanisms studied in a controlled environment. In turn, this helps researchers better understand the mechanisms and processes in disease progression.
By studying the patient’s genes and how they relate to the patient’s illness, scientists can conduct research to find a treatment. This means that doctors can customize each treatment to the patient and their symptoms.
Regenerative medicine
Scientists use iPSCs to grow tissue-specific cells for transplantation. These cells are then given to patients with injuries or degenerative diseases.
In the future, scientists might be able to construct and transplant full organs, not just patches. This could help address the current crisis of organ donor shortages.
Personalized medicine
iPSCs allow for personalized medicine by taking cells from the patient to develop therapies for them.
Since the iPSCs have the patient’s DNA, the patient has a better chance of a positive outcome from treatment. For example, the fact that the iPSC-derived cells have the patient’s DNA, there is less risk of rejection. In turn, this increases the likelihood of treatment success.
Drug discovery
If patients have a disease like diabetes or Astheimers, their DNA codes for the disease. Therefore, any iPSCs created from the patient would also carry the mutation.
Researchers can use these cells to create different tissues, like liver, pancreas, for drug screening to identify possible side effects and effective therapies.
Development
Scientists also use iPSCs to study stages of human development. They differentiate the stem cells into embryonic-stage structures or organoids. This allows researchers to study the molecular process of cells involved in development and organ function.
Limitations of iPSCs
Currently, there’s a lot of excitement about induced pluripotent stem cells, but there are some big challenges to overcome.
One problem is making enough iPSCs and on a large scale in a reliable way. It’s like trying to bake cookies, but it takes a long time, costs a lot, and you only end up with a tiny fraction of the cookies you hoped for.
In the world of stem cells, it takes about 2 to 3 weeks to make iPSCs. Only a very small percentage of cells actually turn into iPSCs successfully.
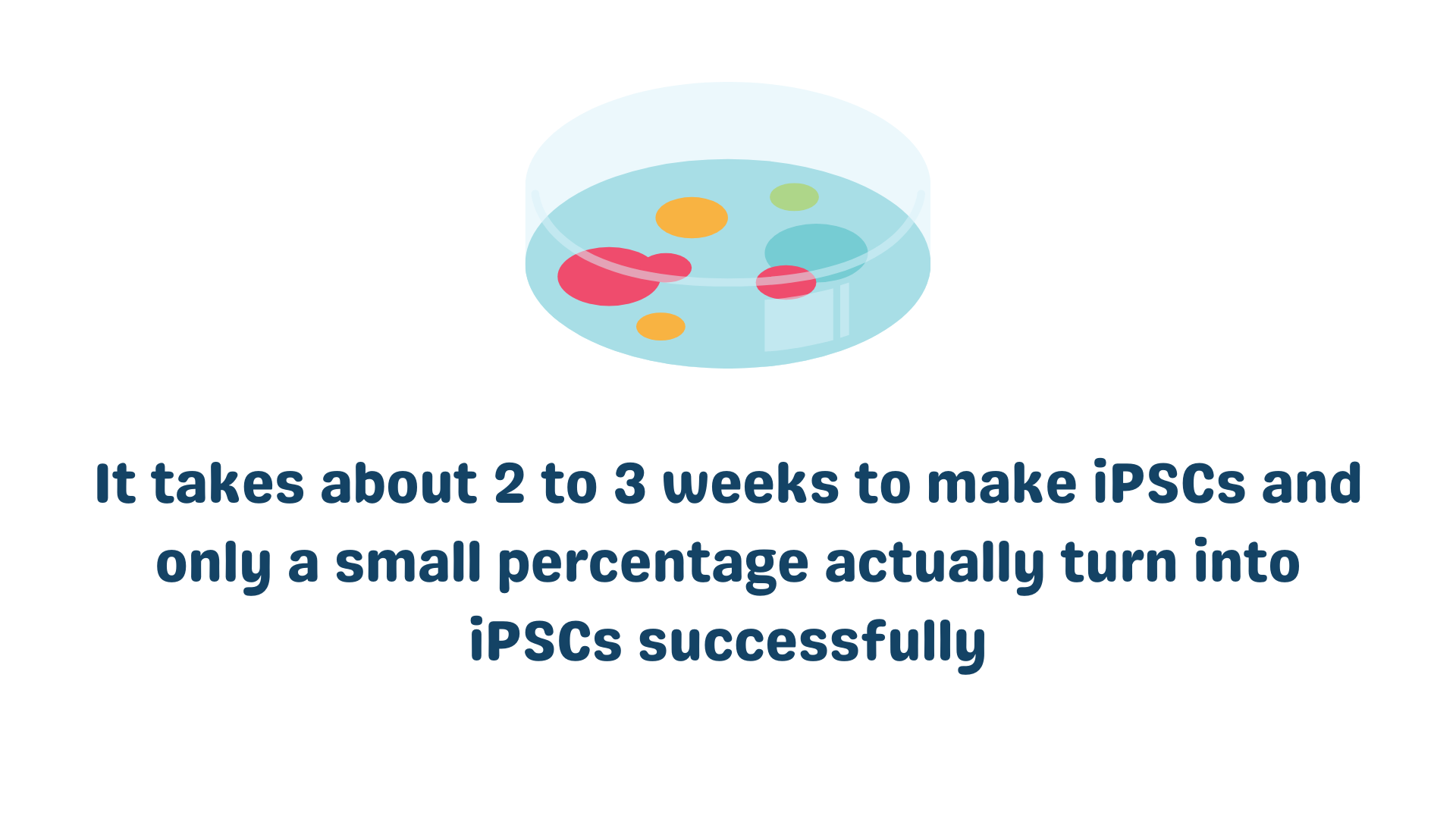
Another challenge is that cells have little “tags” on them. These are like tiny sticky notes that tell them what to do.
When a scientist transforms adult cells into iPSCs, it’s like erasing all those notes and giving the cells a fresh start. But sometimes, the eraser doesn’t work perfectly, and some of those notes are still there.
This means that when a scientist tries to turn iPSCs into specific types of cells, like into heart cells or brain cells, they might still try to act like the original cells they came from.
It’s like if you tried to turn a cookie back into dough and then bake that batch of dough into a new type of cookie. It might not work quite the same way as before. This can make it tricky to use iPSCs for certain treatments or experiments.
Trailhead’s Induced Pluripotent Stem Cells
At Trailhead Biosystems, we’re all about providing scientists with the tools they need to do amazing research. One of our specialties is working with iPSCs from multiple lines and differentiating them using our own protocols.
We’re always working on making iPSCs better and safer to use. We have this cool technology called high-dimensional design of experiments (HD-DoE™), which helps us figure out the best conditions for growing iPSCs and turning them into specific kinds of cells. It’s like a recipe book, but for cells!
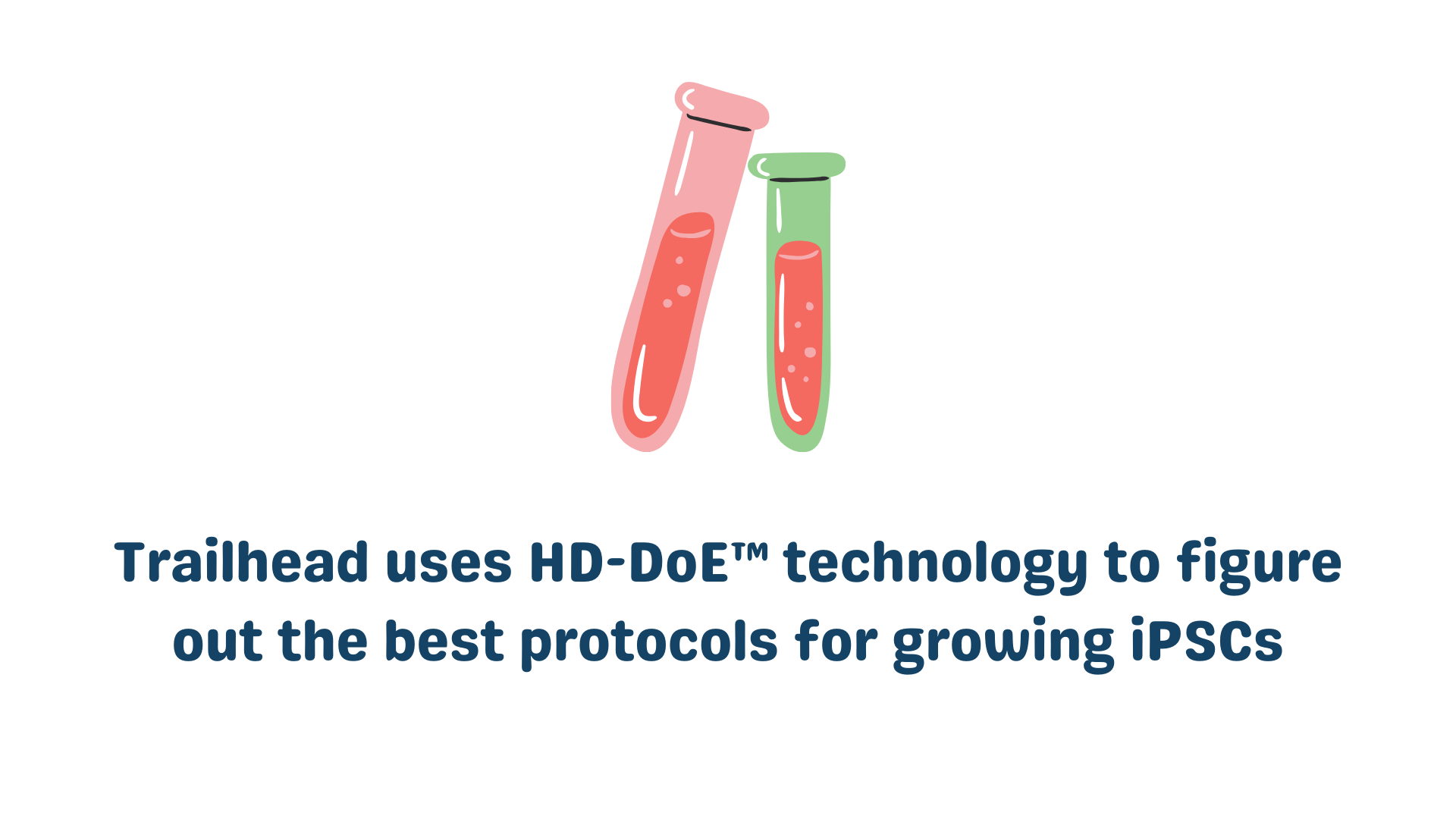
With this technology, we can predict exactly what ingredients and steps the cells need to make iPSCs grow and change into the cells researchers want to study or use for treatments.
Right now, we’re supplying researchers with endothelial cells and pre-myelinating oligodendrocytes. These are important for studying things like blood vessels and the brain.
But we’re not stopping there! Our team is also working hard to figure out how to make other types of cells, like ones that help with forming blood or protecting the brain.
By being able to grow and study these cells in the lab, scientists can learn a lot about how our bodies work and maybe even find new ways to treat diseases. It’s like having a miniature version of the body to experiment with, and it could lead to some really exciting discoveries!
Conclusion
Stem cells are important in the medical field because they can help researchers understand how bodies grow, what goes wrong when people get sick, and even how a doctor might treat diseases.
One type of stem cell, called induced pluripotent stem cells (or iPSCs for short), is especially exciting. They’re made from regular adult cells, but without the ethical and moral dilemmas surrounding the use of embryonic stem cells.
Scientists and doctors believe that iPSCs could change the way we create medicine. Applications for iPSCs include…
- Disease modeling
- Regenerative medicine
- Personalized medicine
- Drug discovery
- Studying human development.
But there’s a catch – differentiating iPSCs isn’t easy. It takes a lot of time and money, and sometimes the process doesn’t work perfectly. But even with these challenges, iPSC-derived cells are a treasure trove of possibilities.
That’s where Trailhead Biosystems comes in. Trailhead aims to overcome these challenges using a computational process to optimize differentiation methodologies needed to supply iPSC-derived cells at commercial levels.